Sample chapter
Below is the introduction to Unmaking the Bomb, reproduced with permission of MIT Press. You can download it as a PDF here. The footnotes have not been included below.
1. Introduction
The invention and use of the atomic bomb in 1945 marked the time when humanity developed the means to end civilization within hours. While nuclear weapons have not been used in war since then, threats and preparations to use them and the pursuit of the capability to produce them have cast a terrible shadow over the world. It is hard to see how disaster can be forever forestalled in a world that now has nine states holding nuclear weapons, with additional countries and terrorist groups possibly seeking to acquire them.
Earlier historical epochs were called the Stone Age and the Iron Age. For almost seven decades, the world has lived in a fissile material age. Fissile materials can sustain an explosive nuclear fission chain reaction that releases enormous energy in the form of the blast, heat, and radiation. The energy from such explosions also is used to ignite the nuclear fusion reactions in modern thermonuclear weapons (hydrogen bombs). All nuclear warheads today contain at least a few kilograms of either plutonium or highly enriched uranium (HEU) and often both. If we are to reduce the threat from nuclear weapons, we must deal with the dangers posed by the production, stockpiling, and use of fissile materials.
The first nuclear explosion—the Trinity test carried out by the United States in the Alamogordo Desert in southern New Mexico on July 16, 1945—was a test of the plutonium bomb that was exploded twenty-four days later over Nagasaki. A bomb made from HEU, based on a different design, so simple that it did not require an explosive test, was used against Hiroshima on August 6, 1945. In each of these two bombs about one kilogram of fissile material fissioned, releasing in about one microsecond energy equivalent to the explosion of nearly 18,000 tons of high explosives.
When he learned of the Hiroshima bombing, Harry Truman, the president of the United States, described it as “the greatest thing in history.”
In his public announcement, he described the weapon as “a new and revolutionary increase in destruction,” with “more than two thousand times the blast power of the . . . largest bomb ever yet used in the history of warfare.” He identified Oak Ridge (Tennessee) and Richland (Washington) as the sites where the United States had “been making materials to be used in producing the greatest destructive force in history.”
Truman also made public the enormous scale, secrecy, and cost of the effort of making the fissile materials for the bombs:
We now have two great plants and many lesser works devoted to the production of atomic power. Employment during peak construction numbered 125,000 and over 65,000 individuals are even now engaged in operating the plants. Many have worked there for two and a half years. Few know what they have been producing. They see great quantities of material going in and they see nothing coming out of these plants, for the physical size of the explosive charge is exceedingly small. We have spent two billion dollars on the greatest scientific gamble in history—we won.
The huge secret effort described by President Truman, code-named the Manhattan Project, was the first successful production of fissile materials in sufficient quantity for a nuclear weapon. Even today, seven decades later, that remains the most significant challenge to acquiring nuclear weapons.
From the outset of the fissile material age prominent scientists had warned of the grave dangers that these new materials and nuclear weapons posed to the world (figure 1.1). In January 1946, in its first resolution, the General Assembly of the newly formed United Nations called for plans for the elimination of nuclear weapons and for control of atomic energy to ensure it was used only for peaceful purposes. Competing plans were developed by the United States and Soviet Union but Cold War suspicions prevented agreement.
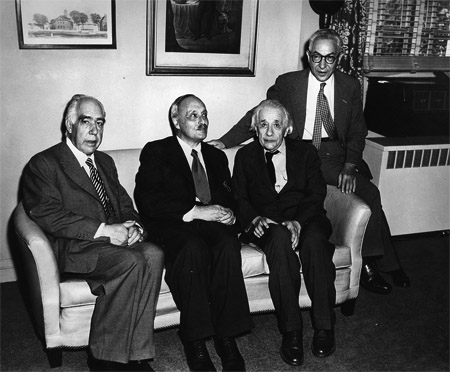
Figure 1.1: Seven decades ago, scientists began warning of the dangers of fissile materials and their use in nuclear weapons. From left: Niels Bohr in 1944 cautioned that fissile materials could become a “perpetual menace” to humankind;a James Franck in 1945 led a group of Manhattan Project scientists in arguing that “the development of nuclear power is fraught with infinitely greater dangers than were all the inventions of the past”; Albert Einstein in 1955 joined philosopher Bertrand Russell and others to issue the Russell-Einstein manifesto calling for the abolition of nuclear weapons and launching the Pugwash movement of scientists for nuclear disarmament; Isidor Rabi in 1949 advised the United States government not to pursue thermonuclear weapons since “The fact that no limits exist to the destructiveness of this weapon makes its very existence and the knowledge of its construction a danger to humanity as a whole.” Photo: Princeton, NJ, October 3, 1954.
Since the failure of the first efforts to ban nuclear weapons and control the use of fissile materials, nine other states have successfully followed the United States and produced nuclear weapons through the uranium enrichment or plutonium separation routes to the bomb. In most cases, weapon states have pursued production of both HEU and plutonium.
The physical and chemical technologies that allow uranium enrichment and plutonium separation also have been mastered by many other states that have considered but decided not to build nuclear weapons. These technologies are now within the reach of a growing number of states with modest scientific and industrial capacity. As fissile material
production technologies spread, so do the opportunities for the spread of nuclear weapons to more countries.
Fissile material production still appears an unlikely route to the bomb, however, for nonstate groups that may be motivated to carry out nuclear terrorist attacks. Such groups would more plausibly try to acquire an existing weapon or stocks of fissile material from which they could fabricate a nuclear explosive—a powerful additional reason for states to seek the elimination of nuclear weapons and fissile material stocks.
At the end of World War II, the United States was the only country with nuclear weapons, and the amount of highly enriched uranium and plutonium that it had produced was on the order of a hundred kilograms in total—enough for only a few nuclear explosives. During the Cold War, the number of nuclear weapons grew to the tens of thousands and the size of stockpiles of fissile materials grew to millions of kilograms (thousands of tons—throughout this book, tons refer to metric tons, with one metric ton corresponding to 1,000 kg or about 2,205 pounds). Globally, the number of nuclear warheads peaked in the late 1980s at over 65,000. To make possible such large numbers of nuclear warheads, the weapon states collectively produced for weapon purposes over 2,000 tons of HEU and about 250 tons of separated plutonium.
With the end of the Cold War, the number of nuclear weapons has declined and is likely to fall further. Even so, in 2013 there were still about 17,000 nuclear warheads worldwide, with almost half of them in line to be dismantled over the next few decades. More than 90 percent of these warheads are held by the United States and Russia. The United Kingdom, France, China, Israel, India, Pakistan, and North Korea have about 1,000 operational warheads between them and perhaps several hundred that have been withdrawn from service. South Africa dismantled its nuclear weapons in the 1990s and joined the Non-Proliferation Treaty as a nonnuclear weapon state.
The dismantlement of tens of thousands of Cold War era warheads has left the weapon states with large stockpiles of excess fissile material, which, if not eliminated, could be used for weapons again. Making nuclear weapons reductions irreversible requires disposing of this fissile material and ending the production of fissile material for weapons in those few countries where it is still going on.
The problem is larger than this, however. Some countries use (or plan to use) plutonium in civilian nuclear power reactors to make electricity, and use highly enriched uranium in civilian and military nuclear research reactors and for military naval propulsion. The largest stockpile of fissile material associated with such activities is the civilian plutonium separated from power reactor spent fuel. All of this material is weapon-usable. To reliably assure against its use for weapons will require an end to the production and use of fissile materials for reactor fuels and the disposal of existing stocks currently earmarked for such fuels.
The goal of this book is to lay out the technical basis for practical policy initiatives that would step-by-step cap, reduce, and eventually eliminate the global stockpile of about 1,900 tons of weapon-usable fissile material including material in weapons or recovered from dismantled weapons, the plutonium used in civilian nuclear power programs, and the HEU in military and civilian research and naval reactor stockpiles. Such initiatives are critical to support deep reductions and ultimate elimination of all nuclear warheads, to make disarmament more difficult to reverse, to raise the barriers to nuclear weapon proliferation to countries that do not have them, and to prevent possible nuclear-weapon acquisition by terrorist groups. They complement the traditional nuclear arms control and nonproliferation agenda, which has focused on capping and reducing deployed nuclear warheads and delivery systems, limiting the testing of such weapons, and international monitoring of civilian nuclear energy programs to deter and detect their potential use for weapons.
How the Nuclear World Emerged
Part I of this book, comprising the next four chapters, provides essential background for understanding the fissile material problem. We summarize this background briefly below.
In chapter 2, we describe the processes for producing fissile materials, how these materials are used in nuclear weapons, and the current national and global stockpiles.
The splitting or “fission” of heavy uranium nuclei was discovered just before World War II, in December 1938, in Germany. Not long afterward, in March 1940, two refugee physicists, Otto Frisch and Rudolf Peierls at the University of Birmingham, England, wrote a technical memorandum alerting the British government that an explosive nuclear fission chain reaction might be possible in a small mass of nearly pure uranium-235. (Natural uranium consists of 0.7 percent uranium-235 and 99.3 percent uranium-238.) They also noted that “effective methods for the separation of isotopes have been developed recently” that could allow uranium-235 separation from natural uranium on a sufficiently large scale to permit construction of an atomic bomb. When it later came to the attention of U.S. scientists and policy makers, this memo galvanized the U.S. bomb program.
While the U.S. effort to design the atomic bomb, led by J. Robert Oppenheimer at Los Alamos, has captured most attention from historians, the largest investment of resources and people in the Manhattan Project was the effort in Oak Ridge, Tennessee, to separate the minor chain-reacting isotope uranium-235 from natural uranium. Three different techniques were developed, but the one that was adopted at the end of the war was gaseous diffusion. This involves the passage of gaseous uranium hexafluoride through thousands of porous barriers with the stream becoming slightly more enriched in uranium-235 at each stage, because the molecules carrying the lighter uranium-235 atoms pass through the barriers slightly more quickly. Gaseous diffusion facilities became enormous and consumed vast amounts of power.
Modern enrichment plants rely on a much more efficient isotope separation technology, gas centrifuges. A gas of uranium hexafluoride is spun at high speed inside a long vertical cylinder so that the molecules carrying the heavier uranium-238 atoms are pressed more tightly against the wall; combined with an axial circulation of the gas in the centrifuge rotor, this process can be used to extract two streams of uranium from the machine, one slightly enriched and one depleted in uranium-235. By connecting many such centrifuges in series and parallel, uranium can be enriched to any desired level, including to “weapon-grade,” which contains more than 90 percent uranium-235.
In 1941, a second element able to undergo a fission chain reaction was discovered, plutonium. Unlike uranium-235, plutonium does not exist in significant concentrations in nature. It is produced by the capture of neutrons by uranium-238 nuclei in a nuclear reactor. The first reactor was built under the leadership of Enrico Fermi at the University of Chicago in December 1942 as part of the Manhattan Project, and the first reactors designed to produce plutonium on a significant scale were built on the Columbia River in central Washington State. According to General Leslie Groves, the man in charge of the Manhattan Project, an isolated location for the production reactor was chosen because “no one knew what might happen, if anything, when a chain reaction was attempted in a large reactor.” One fear was “some unknown and unanticipated factor” might lead a reactor “to explode and throw out great quantities of highly radioactive materials into the atmosphere.”
Separation of plutonium from neutron-irradiated uranium can be done chemically but requires a “reprocessing plant” in which all operations are performed behind thick concrete radiation shielding. Once the plutonium is separated from highly radioactive fission products, however, it can be handled relatively easily and could potentially be made into nuclear weapons, even by a subnational group. This is why proposals made to separate plutonium out of spent power reactor fuel for recycle in nuclear fuel have become so controversial.
The Manhattan Project developed different types of nuclear-weapon designs. A simple but very inefficient “gun-type” design was developed for highly enriched uranium and a much more difficult but efficient “implosion” design was developed for plutonium when it became apparent that the gun-type design would not work for this element. The Hiroshima bomb contained about 60 kilograms of highly enriched uranium while the Nagasaki bomb, which had about the same explosive energy, contained only 6 kilograms of plutonium. Modern versions of the Nagasaki design can use less than 4 kilograms of plutonium or 12 kilograms of highly enriched uranium.
Modern thermonuclear weapons use a “primary” fission explosion to trigger a much more powerful “secondary” explosion involving the fusion of the nuclei of heavy hydrogen atoms. These weapons generally contain both plutonium and highly enriched uranium—on average about 3–4 kilograms of plutonium in the fissile “pit” of the fission primary and 15–25 kilograms of highly enriched uranium in the fission-fusion secondary.
The current nine nuclear weapon states all followed different paths to weapons but in most cases relied on the example and even direct assistance of others. The histories of fissile material production for weapons by these countries are described in chapter 3. The first and most important case is that of the United States. The Manhattan Project, which included in its technical leadership a cadre of European émigré and refugee scientists, pioneered large-scale deployment of the technologies of uranium enrichment and plutonium production and provided a technological roadmap for most of the nuclear weapon programs that followed. The Soviet Union in particular patterned its first fissile material production facilities and its first weapon design on those of the United States. Later, in the early 1960s, the Soviet Union broke new ground by developing and deploying gas centrifuge uranium enrichment technology on a large scale.
Britain’s nuclear weapons program was led by physicists who had participated in the U.S. wartime program. France’s fissile material production complex, which was built in the early 1950s, followed Britain in its choice of technologies and scale. Outside information also was important for China, the first country to acquire nuclear weapons without an advanced scientific and industrial base. Many of China’s nuclear experts were trained in the Soviet Union, which also provided expert advisors and designs for China’s fissile material production facilities. The Soviet experts were withdrawn before China’s uranium enrichment and plutonium production facilities were completed, however, and there were delays as China struggled to complete them.
Israel received secret assistance from France, which provided a complete plutonium production complex, namely, both a nuclear reactor and a reprocessing plant. Faced with a uranium shortage, Israel also engaged in nuclear cooperation and trade with South Africa, receiving uranium from South Africa for Israel’s plutonium-production reactor.
India, the seventh state to acquire nuclear weapons, initially claimed to be interested only in nuclear power when it sought assistance in building nuclear facilities but made a point of keeping its options open. It eventually extracted plutonium from the fuel of a research reactor provided to it for peaceful purposes by Canada and the United States and used the plutonium to make nuclear weapons. For its part, Pakistan took advantage of the growing number of international civilian nuclear technology suppliers in the 1970s—especially those based in Europe—to purchase key components and materials for its gas centrifuge program. Pakistan also received direct assistance from China, including the design of a tested warhead.
In North Korea, the most recent state to have developed and tested nuclear weapons, the Yongbyon plutonium-production reactor is based on the published design of a 1950s era reactor developed by the United Kingdom. Its uranium enrichment program, revealed in 2010, is based on technology transferred from Pakistan in the 1990s. It is possible that North Korea has used this capability to make highly enriched uranium for weapons.
The final case is that of South Africa, which produced nuclear weapons of the gun-type design using highly enriched uranium produced by an inefficient aerodynamic uranium isotope separation process. Since South Africa gave up its nuclear weapons in 1991, the HEU recovered from them has been stored under international monitoring.
Chapter 4 provides an overview of the current global stockpile of roughly 1,900 tons of highly enriched uranium and plutonium categorized by current or intended use. These categories include fissile material in or committed to weapons, in naval nuclear propulsion programs, civilian material, and weapon material that has been declared excess for military purposes and is intended to be used in reactor fuel or disposed of some in some other way.
The largest stockpiles both of HEU and plutonium are held by Russia, followed by those of the United States. There are significant uncertainties in estimates of most of the military stockpiles (typically of the order of 20–30 percent), since only the United States and the United Kingdom have so far made public declarations of their military HEU and plutonium inventories. The uncertainty in the estimate of Russia’s stockpile of HEU is on the order of 100 tons
These estimates make clear, however, that most of the global fissile material stockpile is in military complexes and is overwhelmingly allocated for weapon purposes, amounting to about 935 tons of HEU and almost 140 tons of plutonium. Because of the dismantlement of excess Cold War weapons by Russia and the United States, these stocks are far larger than needed for the actual current warhead stockpiles held by the nuclear weapon states.
A second stock of military fissile material is the almost 180 tons of HEU allocated for naval propulsion reactors, mostly by the United States. Additionally, there are the roughly 260 tons of plutonium separated by several civilian nuclear energy programs. In terms of nuclear weapon equivalents, this plutonium stockpile by itself is sufficient for more than 30,000 nuclear warheads. Finally, about 60 tons of HEU are dedicated to civilian research reactors. While this is the smallest category of fissile material, it is spread across the largest number of states and has been the focus of the most strenuous efforts to make it more secure and to reduce the number of facilities in which it is stored.
The uncertainties in estimates of the global fissile material stockpile are equivalent to several thousand nuclear warheads. As nuclear arsenals are further reduced, uncertainties of such magnitude could become obstacles to progress toward nuclear disarmament. Greatly increased nuclear transparency, including the development of new bilateral and multilateral cooperative approaches to verify declarations by states of their fissile material production histories, will be required to reduce the uncertainties in estimated national fissile material inventories and build international confidence to support deeper cuts in the nuclear arsenals. In addition to an examination of production records, this verification will include techniques of nuclear archaeology, which involve the use of physical measurements at shutdown fissile material production facilities to independently estimate historical production at the facilities.
The Nuclear Weapons–Nuclear Energy Link
Along with the emergence of the nuclear weapon states, there was a spread of civilian nuclear technology to scores of countries, impelled in significant degree by the U.S. and Soviet Atoms for Peace initiatives launched in 1953. Atoms for Peace also led in 1957 to the establishment of the International Atomic Energy Agency (IAEA) with a mandate both to promote peaceful uses of nuclear technology and to monitor these uses to assure that fissile materials are not diverted to weapons use. This approach to managing the diversion risks of civilian nuclear energy programs was codified in the Treaty on the Non-Proliferation of Nuclear Weapons (commonly known as the Non-Proliferation Treaty or NPT) of 1970. Part II of this book addresses the challenges of stopping the spread of nuclear weapons to countries that do not have them and minimizing the risk of terrorist groups getting access to weapon-usable materials.
Almost any state could construct a nuclear device if it obtained the requisite amount of highly enriched uranium or separated plutonium or other less common fissile material. It is also prudent to assume that a terrorist group could make a nuclear explosive device if it had access to fissile material. A 1988 study by J. Carson Mark, for twenty-five years head of the Theoretical Division at the Los Alamos National Laboratory, and four colleagues, including the well-known nuclear weapons designer Theodore Taylor, concluded:
Crude nuclear weapons (similar to the Hiroshima gun-type and Nagasaki implosion-type weapons) could be constructed by a group not previously engaged in designing or building nuclear weapons provided that they have the technical knowledge, experience, and skills in relevant areas, e.g., the physical, chemical, metallurgical and nuclear properties of the various materials to be used, as well as the characteristics affecting their fabrication, and the technology of high explosives and/or chemical propellants.
Efforts to strengthen the barriers to nuclear weapons production therefore must focus especially on increasing the difficulties a country or subnational group would face in acquiring fissile materials. Today, the most plausible route to nuclear weapons for subnational groups would be to obtain separated plutonium or highly enriched uranium in fresh reactor fuel or from a fuel fabrication facility. Eliminating fuels that contain such materials therefore could greatly reduce the danger of terrorist acquisition of nuclear weapons.
With respect to nuclear weapon proliferation by countries, the situation is more complicated because countries could produce as well as divert fissile material. Speaking on the sidelines of the April 2012 Nuclear Security Summit in Seoul, South Korea, U.S. President Barack Obama observed: “The very process that gives us nuclear energy can also put nations and terrorists within the reach of nuclear weapons. We simply can’t go on accumulating huge amounts of the very material, like separated plutonium, that we’re trying to keep away from terrorists.”
Chapter 5 describes the spread of nuclear technology for civilian purposes initiated by the 1953 Atoms for Peace initiative.9 The worldwide promotion of nuclear power that accompanied the Atoms for Peace programs stimulated interest in developing national nuclear science and engineering communities and industries. Atoms for Peace included the export, mostly by the United States and Soviet Union, of research reactors and highly enriched uranium fuel for them to over forty countries.
Inevitably, a growing number of countries sought to acquire the technologies to enrich uranium for light water reactor fuel and to separate plutonium to fuel plutonium breeder reactors, which the United States and other industrialized countries saw as the reactors of the future. The acquisition of reprocessing and enrichment technologies puts countries just a short step away from nuclear weapons, should they decide to seek them.
Today, there are two principal nuclear “fuel cycles” in use. Most of the thirty or so countries with nuclear power plants, including the United States, which has about one-quarter of the world’s power reactors, use fuel “once-through.” The dominant reactor type, the light water reactor, so called because it is cooled by ordinary “light” water, is fueled by low-enriched uranium with about 3–5 percent uranium-235. The discharged spent fuel is stored pending its final disposal. This once-through fuel system has the critical advantage that nowhere in it is weapon-usable fissile material easily accessible. The low-enriched uranium in the fresh fuel cannot sustain an explosive chain reaction without further enrichment, and the plutonium in the spent fuel is never separated from the highly radioactive products of uranium fission. This effectively eliminates the chances of a subnational group acquiring fissile material from this fuel cycle.
The principal proliferation concern about countries using low-enriched uranium fuel once through is that a national enrichment plant designed to produce the low-enriched uranium could be converted rapidly to produce weapon-grade uranium. This possibility has been at the heart of international anxiety about Iran’s uranium enrichment program, which has been producing material enriched to less than 5 percent uranium-235 for possible power reactor fuel as well as a smaller amount enriched almost to 20 percent uranium-235 for use in the Tehran Research Reactor.
The second prevalent nuclear fuel cycle is used primarily in France, which operates the second largest number of power reactors. It involves the separation of plutonium from the spent reactor fuel at a reprocessing plant and its recycle in reactor fuel, either as a mixed uranium plutonium oxide (MOX) fuel in light water reactors or as fuel for breeder reactors.
From the earliest days of the nuclear era, interest in civilian reprocessing was driven by the dream of breeder reactors that would produce more chain-reacting material than they consumed, typically by converting non-chain-reacting uranium-238 into plutonium. One legacy of this effort to commercialize breeder reactors is the global stockpile of 260 tons of civilian plutonium. Chapter 6 focuses on ending the separation of plutonium for reactor fuel.
Breeder reactors have been plagued by high capital costs and reliability problems. Despite over $100 billion spent over a period of fifty years in over half a dozen countries on breeder reactor research, development, and demonstration, commercialization efforts largely failed. Ambitious breeder development programs in the United States, the United Kingdom, and Germany were abandoned in the 1980s and 1990s. France and Japan have postponed their breeder programs. Today, only Russia and India—recently joined by China on a pilot scale—separate plutonium with the intention of using it as fuel for prototype plutonium breeder reactors.
While the United States gave up both on breeder reactors and on reprocessing in the early 1980s, some other countries continued reprocessing even after postponing breeder commercialization and decided to mix their separated plutonium with uranium in MOX fuel for existing light water reactors. The spent MOX fuel is stored pending future developments. This fuel cycle is more costly than the once-through fuel cycle and also complicates radioactive waste disposal. Most countries that have tried it have therefore abandoned it. In 2012, the United Kingdom decided to end reprocessing when it completed its existing contracts. In France and Japan, as of this writing, the future of reprocessing was in debate but still unresolved.
In the 1970s, India demonstrated that civilian reprocessing opens a route to nuclear weapons for non-weapon states. It also creates a risk of the theft and use of plutonium by subnational groups since, unlike spent nuclear fuel assemblies, separated plutonium has no radiation barrier, making it easy to handle (e.g., in a simple glove box), and less than 8 kilograms are required to make a nuclear weapon. Plutonium oxide also could be used in a radiological dispersal device because it is extremely carcinogenic if inhaled.
Given that the breeder reactors that provided the original justification for the civilian separation of plutonium have proven largely unworkable, unnecessary, and uneconomical, and that separated plutonium is a clear proliferation risk, it would be appropriate to phase out the continued separation and use of plutonium as a fuel.
Chapter 7 assesses the challenges of ending the use of HEU as a reactor fuel. HEU is not used as a power reactor fuel but it is used to fuel a large fraction of the world’s research and naval propulsion reactors. Access to 50–100 kilograms HEU would give even a relatively unsophisticated group the means to build a nuclear explosive device. Manhattan Project physicist and Nobel laureate Luis Alvarez observed that an improvised HEU nuclear explosive device with a possible yield of several kilotons could be as simple as an arrangement for dropping one subcritical mass of HEU onto another to create a supercritical mass.
The U.S.-led Global Threat Reduction Initiative is carrying forward the effort to minimize civilian use of HEU in research reactors by converting them to low-enriched uranium fuel and removing fresh and spent HEU fuel from their sites. Russia has cooperated in this effort in countries to which the Soviet Union provided HEU-fueled research reactors. The U.S. and Russian efforts have successfully cleaned out HEU from about half the more than forty nonnuclear weapon states with HEU and are making progress in the other half. However, Russia has only recently started to give priority to converting or shutting down its own HEU-fueled research reactors, as of 2013, these accounted for more than half of the world’s remaining unconverted facilities.
HEU in naval fuel cycles also is a security risk. The single largest illicit diversion of fissile material may have been the hundreds of kilograms of weapon-grade uranium that were secretly transferred in the 1960s from a naval fuel fabrication facility in the United States to Israel, apparently with the cooperation of the plant’s owner (see chapter 3). In 1993, the theft of a much smaller amount of HEU submarine fuel from a Russian storage facility helped focus attention on the need to secure Russia’s nuclear materials after the collapse of the Soviet Union.
Despite the greater attention given to fissile material security after the attacks of September 11, 2001, significant problems with protecting HEU in storage have surfaced even in the U.S. nuclear weapons complex. In July 2012, three antinuclear activists successfully penetrated the high security system surrounding the newly built Highly Enriched Uranium Materials Facility at the Y-12 site in Tennessee, which contains over 100 tons of highly enriched uranium. The breach was attributed to a range of problems, including both inoperable and poorly functioning security cameras, a failure to react to alarms, and inadequate response plans and procedures at the site. The United States Department of Energy, which manages the nuclear weapons complex, spends about $1 billion a year on physical security of nuclear materials.
Large quantities of HEU are used to fuel the nuclear navies of the United States, Russia, and the United Kingdom, and India planned to begin trials in 2014 of its first HEU-fueled nuclear submarine. The United States alone has reserved 152 tons of weapon-grade uranium for future use in naval propulsion reactors—enough for 5,000–10,000 nuclear explosives. France, however, fuels its submarines and a nuclear-powered aircraft carrier with low-enriched uranium (LEU) fuel. It is believed that China also uses LEU fuel and Brazil, which is planning to be the first non-weapon state to have a nuclear-powered submarine, also has chosen to use LEU fuel—at least initially. If the countries that now use HEU in naval fuel also converted to LEU, much more HEU could be declared excess and eliminated. But this issue has thus far not received much attention.
Eliminating Fissile Materials
The third and final part of this book (chapters 8, 9, and 10) explores how initiatives to cap and reduce fissile material stockpiles could support and even drive progress toward nuclear disarmament. A nuclear weapon-free world would be far more stable if it were also a fissile material-free world. This would involve ending production of fissile materials and eliminating the current global fissile material stockpile in a verifiable and irreversible manner.
Chapter 8 discusses a proposed treaty that would end the production of fissile material for weapons, the Fissile Material Cutoff Treaty (FMCT). Such a treaty would build on the fact that the United States, Russia, the United Kingdom, and France have already declared that they have permanently ended their production of fissile materials for weapons, while China has suspended production. An FMCT has been under consideration at the United Nations Conference on Disarmament in Geneva since 1993, but linkages to other issues—in recent years by Pakistan—have blocked the launch of formal negotiations.
Once negotiations do go forward, the two critical issues will be the scope of the treaty and how it will be verified. The principal question on scope is whether the weapon states will commit permanently and verifiably not to use for weapons preexisting stocks of fissile material that they currently consider excess to their weapons needs.
Much of the verification of an FMCT could be carried out using the same monitoring techniques developed by the IAEA to verify that non-weapon states are complying with their NPT obligation not to use fissile materials to produce “nuclear weapons or other nuclear explosive devices.” The IAEA or (less likely) another inspection agency established by the treaty would monitor enrichment plants in the weapon states to determine whether they are producing HEU and, if they are, monitor the subsequent storage or use of the HEU. Plutonium and other specified fissile materials newly separated at weapon state reprocessing plants would similarly be subject to such international monitoring. And, if it were part of the agreement, the storage and use of preexisting fissile materials that the weapon states have declared excess for all military purposes too would be monitored.
It will be critical under an FMCT to verify that there is no clandestine production of highly enriched uranium or plutonium at undeclared sites. To accomplish this, international inspectors would have to be authorized to take environmental samples within weapon states as well as non-weapon states and to initiate “challenge inspections” at suspect facilities. Analysis of air samples might, for example, be able to detect degradation products of uranium hexafluoride leaked from enrichment operations or elevated levels of krypton-85, a gaseous fission product released by reprocessing.
The most effective and enduring way to deal with the security dangers posed by fissile materials is to dispose of them as irreversibly as possible. Chapter 9 describes current approaches to disposing of highly enriched uranium and alternative options for disposing of plutonium.
The disposal of highly enriched uranium is relatively straightforward. It is blended with natural or slightly enriched uranium to produce low-enriched uranium that can then be used as a fuel for light water nuclear power reactors commonly used to produce electricity. As of 2013, Russia and the United States have already down-blended a combined total of over 600 tons of highly enriched uranium, most dramatically with 500 tons of Russian weapon-grade uranium blended down for fuel for U.S. reactors under a U.S.-Russian deal concluded in 1993.
The disposal of weapons plutonium has proven costly and complicated and has made little progress. Although the United States and Russia concluded in 2000 a Plutonium Management and Disposition Agreement that committed each party to dispose of at least 34 tons of weapon-grade plutonium “withdrawn from nuclear weapon programs,” this process is currently not scheduled to begin until 2018. The United States has planned to fabricate most of its excess plutonium into MOX fuel for use in light water reactors but, as of 2013, the future of this program was in doubt.
Irradiating plutonium in a light water reactor as MOX fuel eliminates a fraction of the initial material. The remaining plutonium survives but becomes encapsulated in a highly radioactive spent fuel matrix similar to the one from which it was first separated. The plutonium could not be used for weapons without once again separating it from the spent fuel in a reprocessing plant. The spent MOX fuel would be disposed of in a geological repository. As MOX program costs have spiraled and delays mounted, the United States has decided to consider alternative means of disposal of its excess weapons plutonium.
Russia plans to use its excess separated plutonium in prototype breeder reactors. As with the MOX option, the spent fuel still contains plutonium. Russia intends to eventually separate the plutonium and use it again as reactor fuel, creating in effect a perpetual river of separated plutonium.
Nonreactor options for plutonium disposal merit greater attention than they have received. One is to mix the plutonium back into the concentrated fission-product wastes from which it was originally separated. This plutonium-bearing waste could then be placed in a deep geological repository with regular spent nuclear fuel. Another option would be to immobilize the plutonium in a durable matrix and dispose of it underground in 3–5 kilometer deep boreholes.
Chapter 10 offers a summary of the fissile material perspective on nuclear disarmament and makes a case for three policy goals that could reduce the danger from today’s large global stock of fissile material and nuclear weapons.
1. Radically increase the transparency of nuclear warhead and fissile material stockpiles to allow for verified deep reductions in these stockpiles as part of the process of nuclear disarmament.
2. Verifiably end all further production and use of HEU and plutonium as fuel both for civilian and military reactors.
3. Verifiably eliminate military and civilian fissile material stockpiles as irreversibly as possible.
Confidence in and verification of nuclear disarmament will be far easier in a world where there is no production or use of separated plutonium or highly enriched uranium and where fissile material stocks have been eliminated. More drastically, if civilian nuclear power were phased out in parallel with nuclear weapons, it would become more difficult and more time-consuming for any country to make fissile materials for nuclear weapons and would make it easier for the international community to detect and respond to what would be a clear threat to international peace and security.